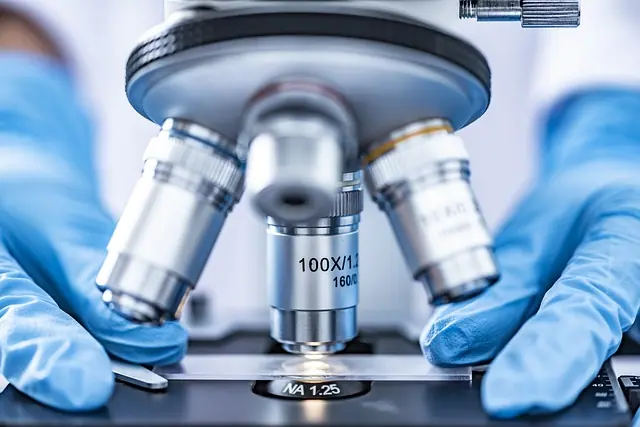
Everything You Need to Know About Synthetic gRNA
Advances in genetic engineering have revolutionized the area of biotechnology in recent years. The invention of synthetic guide RNA (gRNA), a critical component of the CRISPR-Cas9 gene-editing system, is one such achievement. Synthetic gRNA allows for unparalleled accuracy and efficiency in modifying genetic material, giving researchers a powerful tool for studying and perhaps treating a wide range of diseases.
This article will look at the fundamentals of synthetic gRNA, including its mechanism of action, uses, and future possibilities. We will delve into its synthesis, delivery methods, design considerations, and potential ethical implications.
Whether you are a student, scientist, or simply inquisitive about cutting-edge genetic engineering science, this thorough tutorial will give you an essential understanding of synthetic gRNA.
The Basics of Synthetic gRNA
A synthetic guide RNA (gRNA) molecule is a single-stranded RNA molecule designed to direct the CRISPR-Cas9 system to a specific target location in the genome. It is a molecular tool that guides the Cas9 enzyme to the appropriate DNA region for precise gene editing. By attaching to the target DNA, synthetic gRNA allows Cas9 to induce double-stranded breaks, therefore commencing the DNA repair process.
Structure and Components
The scaffold and the target-specific sequence are two essential components of gRNA. The scaffold is a stable structure containing the components required to bind successfully with Cas9, a critical enzyme in gene editing. Conversely, the target-specific sequence is essential in selecting the precise position for gene editing. Designers typically create the target-specific sequence, which is usually 20 nucleotides long, to complement the precise region of DNA undergoing modification.
Mechanism of Action
The mechanism through which synthetic gRNA works follows a systematic procedure.
- Initially, gRNA binds to the Cas9 enzyme, forming a complex.
- This complex is directed towards the DNA sequence that matches the gRNA using the target-specific sequence, producing a DNA-RNA hybrid.
- The Cas9 enzyme subsequently breaks the double-stranded DNA. After this, the cell’s DNA repair mechanisms, such as non-homologous end joining (NHEJ) or homology-directed repair (HDR), step in to repair the DNA breaks.
- Finally, these repair pathways result in changes to the targeted gene, completing the intended gene editing.
Synthetic gRNA Synthesis
Design Considerations
Several things must be addressed while creating synthetic gRNA. These include specificity, stability, and efficiency. The target-specific sequence should be carefully planned to provide high specificity, eliminating off-target locations. Furthermore, the scaffold area must maintain structural integrity to promote Cas9 binding and avoid degradation.
Chemical Synthesis Methods
- Solid-Phase Synthesis: A common approach for chemically synthesizing gRNA is solid-phase synthesis. It entails adding nucleotides to a solid support in a sequential manner, often utilizing phosphoramidite chemistry. The expanding RNA chain is formed in the 3′ to 5′ orientation by linking protected nucleotides one at a time. Following synthesis, the gRNA is deprotected and cleaved off the solid support, resulting in a chemically synthesized gRNA molecule.
- Enzymatic methods: Enzymatic techniques can also be used to produce gRNA. T7 polymerase-based transcription is one such approach. A DNA template with a T7 promoter region is employed in this method. T7 polymerase recognizes the T7 promoter and begins transcription, resulting in the synthesis of gRNA. This method is useful for large-scale manufacturing since it allows easy scaling and gRNA purification.
In Vitro Transcription
In vitro transcription (IVT) is an alternate approach for creating sgRNA that includes transcribing the sgRNA from a matching DNA template outside of the cell. The procedure begins with creating a DNA template containing the guide sequence and an RNA polymerase promoter site upstream of the sgRNA sequence. After obtaining the DNA template, the sgRNA is transcribed using recombinant RNA polymerase kits and other reagents.
The IVT method normally takes 1-3 days to synthesize gRNA. This strategy, however, has several downsides. Using in vitro-transcribed sgRNA in CRISPR investigations often requires additional purification due to its tendency to be time-consuming and prone to mistakes. Because of these constraints, editing efficiency might vary, and the potential of off-target consequences increases.
Furthermore, IVT sgRNA can be harmful to some cell types. Several studies have shown that IVT sgRNA may induce innate immune responses in human and mouse cells, resulting in cytotoxicity and death. This reaction is thought to be triggered by the 5′ triphosphate group found in IVT sgRNAs, which is recognized by DDX58, a cytoplasmic innate immune response receptor. DDX58 then stimulates the synthesis of type I interferons and proinflammatory cytokines, resulting in cell death.
Applications of Synthetic gRNA
1. Research and Biotechnology
Synthetic gRNA has reshaped science and biotechnology by giving a potent tool for precise gene editing. One of the most common uses is gene knockout, where researchers introduce disruptive mutations into target genes to examine their function and reveal disease mechanisms. Researchers can deduce the gene’s significance in biological processes by inactivating certain genes and observing the subsequent phenotypic changes.
Knock-in experiments are also possible with synthetic gRNA, allowing the insertion of particular DNA sequences or changes at targeted genomic sites. This method is extremely useful for researching gene expression, protein localization, and functional analysis.
2. Therapeutic Potential
Synthetic gRNA has enormous therapeutic potential, notably in gene therapy. This gRNA can accurately target disease-causing genes and make therapeutic alterations by utilizing CRISPR-Cas9 technology. Synthetic gRNA can repair the genetic sequence and restore normal gene function in genetic illnesses caused by mutations. It also allows for introducing therapeutic genes into particular cell types, possibly addressing illnesses caused by genetic deficiencies.
Clinical studies are underway to assess the safety and efficacy of synthetic gRNA-based treatments. In treating genetic disorders such as sickle cell disease and beta-thalassemia, where CRISPR-Cas9-mediated gene editing is being investigated as a potential therapy, promising findings have been reported.
3. Agricultural and Environmental Applications
Aside from human health, synthetic gRNA can revolutionize agricultural and environmental management. In agriculture, we can use it to enhance agricultural attributes by changing genes associated with yielding, pest and disease resistance, and nutritional content. Researchers, for example, are employing synthetic gRNA to create crops with improved nutritional profiles, reduced vulnerability to diseases, and better tolerance to environmental stresses.
Conclusion
Synthetic gRNA has emerged as a revolutionary tool in genetic engineering, allowing for the precise and efficient modification of genetic material. The design, synthesis, and delivery methods have rapidly advanced, broadening their uses in research, biotechnology, and possible medicinal treatments. Understanding the fundamentals of synthetic gRNA allows researchers and enthusiasts to safely harness its potential and contribute to significant discoveries and improvements.
As technology advances, synthetic gRNA has the potential to open new doors in the fields of healthcare, agriculture, and environmental conservation. The future of genetic engineering offers enormous promise, and synthetic gRNA is at the front of this transformation.